Epigenetic alterations are changes in gene expression that do not involve changes in the DNA sequence itself, but rather modifications to the chromatin structure that affects how the DNA is packaged and expressed. These alterations can have a significant impact on metabolism and can contribute to negative long-term effects on health. Here are some examples:
- DNA methylation: Perhaps the most well-known epigenetic alteration. This is a common epigenetic modification that involves the addition of a methyl group to a cytosine base in the DNA. DNA methylation can affect the expression of genes involved in metabolism, including those involved in insulin signaling and lipid metabolism. Aberrant DNA methylation patterns have been associated with metabolic disorders such as obesity and type 2 diabetes.
- Histone modifications: Histones are proteins that help package DNA into chromatin. Chemical modifications to histones can affect the structure of chromatin and the accessibility of genes for transcription. Histone modifications have been linked to metabolic disorders such as obesity and insulin resistance.
- Non-coding RNAs: Non-coding RNAs (ncRNAs) are RNA molecules that do not code for proteins, but instead regulate gene expression by interacting with DNA or other RNAs. ncRNAs have been implicated in the regulation of metabolism and the development of metabolic disorders.
- Mitochondrial epigenetics: Mitochondria are organelles that generate energy for the cell. They have their own genome, which is subject to epigenetic modifications. Mitochondrial epigenetic alterations have been associated with metabolic disorders such as obesity and insulin resistance.
Overall, epigenetic alterations can have significant impacts on metabolism and contribute to the development of metabolic disorders such as obesity and type 2 diabetes.
DNA Methylation
DNA methylation is an epigenetic modification that involves the addition of a methyl group to a cytosine base in the DNA. DNA methylation can have various impacts on cellular health, DNA stability, and telomere attrition, and can also play a role in cellular senescence and mitochondrial dysfunction.
First, DNA methylation can affect the expression of genes and, therefore, impact cellular health. Methylation of genes involved in cellular processes such as DNA repair, apoptosis, and cell cycle regulation can lead to dysfunction and potentially promote the development of cancer.
Second, DNA methylation can affect DNA stability by promoting mutations and chromosomal rearrangements. This can lead to genomic instability and increase the risk of developing cancer and other diseases.
Third, DNA methylation can impact telomere attrition, which is the gradual shortening of the protective caps at the ends of chromosomes that occurs with each cell division. Telomere shortening can contribute to cellular aging and senescence. Methylation of the telomerase reverse transcriptase (TERT) gene has been shown to reduce telomerase activity and promote telomere shortening.
Finally, DNA methylation can also impact cellular senescence and mitochondrial dysfunction. Cellular senescence is a state of irreversible cell cycle arrest that occurs in response to various stressors, including DNA damage. DNA methylation has been implicated in the regulation of genes involved in the senescence process. Additionally, DNA methylation in mitochondrial DNA (mtDNA) has been associated with mitochondrial dysfunction, which can contribute to a range of diseases, including cancer, neurodegenerative disorders, and metabolic disorders.
DNA methylation is an epigenetic modification that can impact cellular health, DNA stability, telomere attrition, cellular senescence, and mitochondrial dysfunction, virtually all of the hallmarks of aging. Its effects are complex and context-dependent, and further research is needed to fully understand its role in health and disease. Measuring DNA methylation is important to get a sense of the pace of aging of an individual. However, different cells age at different rates so a lot of testing is required.
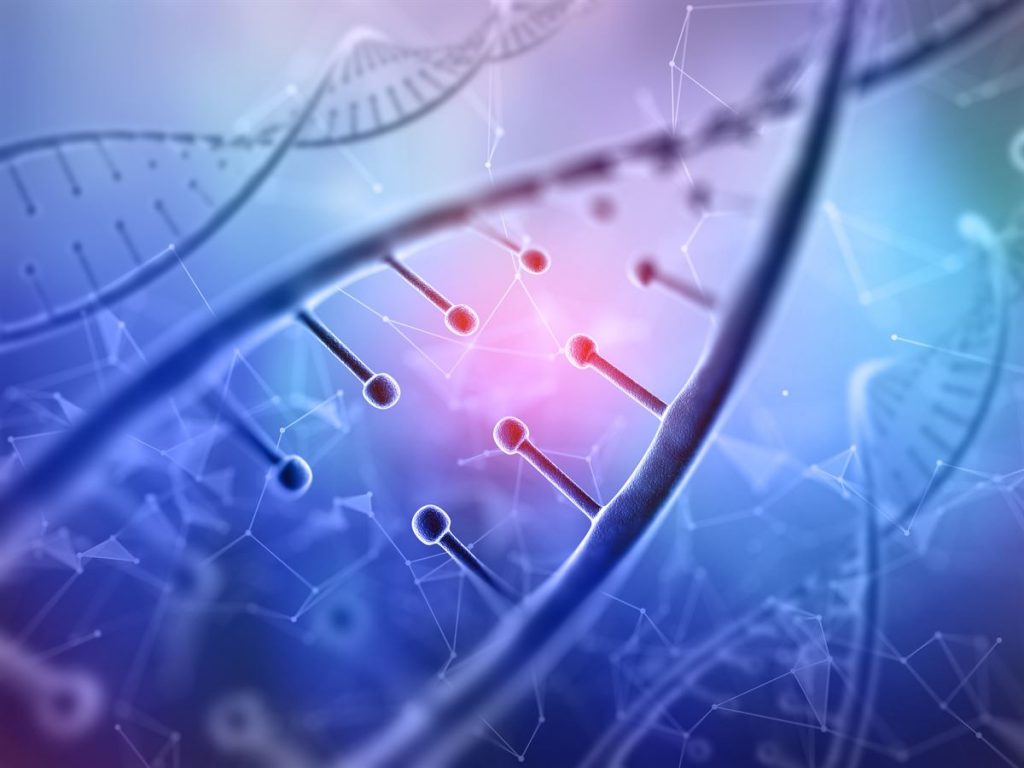
DNA methylation has a lot of potential as a way of measuring healthspan, but a lot of research is still required.
Histone modifications
Histones are proteins that package DNA into chromatin, the complex structure that makes up chromosomes. Histone modification is an epigenetic alteration that involves chemical modifications to the histone proteins that affect chromatin structure and the accessibility of DNA to transcription factors and other regulatory molecules.
Histone stability is important for the DNA to be regulated and transcripted perfectly and modifications to the histones can affect many aspects of health.
Histone modification can impact health in several ways, including DNA stability and replication accuracy. For example, histone modifications can affect the accessibility of DNA repair machinery to damaged regions of DNA, which can lead to errors in DNA repair and DNA instability.
Histone modifications have also been linked to metabolic disorders such as obesity and insulin resistance. In particular, histone modifications can affect the expression of genes involved in metabolism, such as those regulating glucose and lipid metabolism. For example, histone modifications have been shown to affect the expression of the gene for adiponectin, a hormone that regulates insulin sensitivity and energy homeostasis. Changes in adiponectin expression have been linked to obesity and insulin resistance.
Additionally, histone modifications can affect the accuracy of DNA replication by regulating the timing and efficiency of replication fork progression. Errors in DNA replication can lead to mutations and genomic instability, which can increase the risk of developing cancer and other diseases.
Overall, histone modification is an important epigenetic mechanism that can impact health by affecting DNA stability, replication accuracy, and the expression of genes involved in metabolism. Dysregulation of histone modification has been linked to a range of diseases, including metabolic disorders and cancer. Further research is needed to fully understand the role of histone modification in health and disease.
Non-coding RNA’s epigenetic alterations
Epigenetic alterations through non-coding RNAs can have significant impacts on the organism, metabolism, and cell function. Non-coding RNAs (ncRNAs) are RNA molecules that do not code for proteins but instead have regulatory functions in gene expression.
One type of ncRNA that can affect epigenetic modifications is called microRNA (miRNA). MiRNAs can bind to specific mRNA molecules and prevent their translation into protein, leading to the downregulation of gene expression. This can impact various metabolic pathways and cellular functions. For example, altered expression of miRNAs has been implicated in the development of metabolic disorders such as obesity, insulin resistance, and type 2 diabetes.
Another type of ncRNA that can affect epigenetic modifications is called long non-coding RNA (lncRNA). LncRNAs can interact with chromatin-modifying complexes and impact epigenetic modifications such as DNA methylation and histone modification. Dysregulation of lncRNAs has been linked to various diseases, including cancer and neurodegenerative disorders.
In addition to miRNAs and lncRNAs, other types of ncRNAs, such as small interfering RNAs (siRNAs) and piwi-interacting RNAs (piRNAs), can also play a role in epigenetic regulation and impact cellular function.
The dysregulation of ncRNAs can lead to aberrant (departing from standard) epigenetic modifications, resulting in altered gene expression and potentially leading to disease states. Therefore, understanding the role of ncRNAs in epigenetic regulation is crucial for advancing our understanding of disease mechanisms and developing potential therapeutic interventions.
Mitochondrial dysfunction and mitochondrial epigenetic alterations
Mitochondria are organelles found in eukaryotic cells that play a crucial role in cellular energy production, regulation of metabolism, and cellular signaling. They contain their own genetic material, mitochondrial DNA (mtDNA), which encodes for some of the proteins necessary for mitochondrial function.
Mitochondrial epigenetics refers to the study of epigenetic modifications that occur in mtDNA and mitochondrial proteins, as well as the impact of these modifications on mitochondrial function and cellular health. Epigenetic modifications in mtDNA and mitochondrial proteins can occur through changes in DNA methylation, histone modification, and the activity of non-coding RNAs as discussed in the sections above.
Alterations in mitochondrial epigenetics can impact cell function in several ways. For example, changes in mtDNA methylation can affect the transcription of mitochondrial genes, leading to altered mitochondrial function and metabolism. Altered mitochondrial functions are rarely good and may lead to weaker metabolism of the cell.
Mitochondria are responsible for the energy regulation of the cell, so whenever something goes wrong at the mitochondrial level, it will reflect on the cell as a whole.
Mitochondrial histone modifications can also impact gene expression and mitochondrial function. Dysregulation of mitochondrial epigenetics has been linked to various diseases, including cancer, metabolic disorders, and neurodegenerative diseases.
Changes in mitochondrial epigenetics can also impact the health of a cell and its replication capability. The accumulation of epigenetic modifications in mtDNA over time has been associated with cellular aging and decreased cellular replication capability. Additionally, mitochondrial dysfunction resulting from altered mitochondrial epigenetics can lead to cell death and contribute to the development of diseases.
Overall, the study of mitochondrial epigenetics is an important area of research for understanding the mechanisms underlying cellular function and disease development.
Positive epigenetic alterations
There is growing evidence that various environmental stressors, such as fasting, exposure to cold temperatures, and mild stress, can induce positive epigenetic alterations that may confer health benefits. Some examples of beneficial epigenetic alterations that have been observed in response to these stressors include:
- Fasting: Fasting has been shown to induce changes in DNA methylation and histone modification patterns that are associated with improved metabolism and reduced risk of age-related diseases. For example, studies in animals and humans have found that fasting can increase the expression of genes involved in autophagy (a process by which cells recycle damaged or dysfunctional components) and reduce inflammation.
- Cold exposure: Exposure to cold temperatures, also known as cold stress, can induce epigenetic changes that promote thermogenesis (heat production) and improve metabolic function. For example, studies have found that cold exposure can increase the expression of genes involved in brown adipose tissue (BAT) activation, which plays a key role in thermogenesis and energy expenditure.
- Mild stress: Exposure to mild stressors, such as exercise or exposure to low doses of toxins, can induce epigenetic changes that improve cellular function and protect against disease. For example, studies have found that exercise can induce changes in DNA methylation and histone modification patterns that are associated with improved metabolism, cardiovascular function, and brain function.
These stressors and other stimuli can induce beneficial epigenetic alterations that may promote health and protect against disease. However, it is important to note that the specific epigenetic changes induced by these stressors may vary depending on the individual and the duration and intensity of the stressor. Further research is needed to fully understand the epigenetic mechanisms underlying the health benefits of these stressors.
Pharmacological interventions for epigenetic alterations
Pharmacological and environmental longevity interventions impacting epigenetic mechanisms. A number of pharmacological substances mediate their beneficial effects on life span via epigenetic enzymes or upstream regulators that influence levels of crucial metabolites. Caloric restriction was demonstrated to positively affect life span acting via similar mechanisms, while some amino acids and vitamins support the generation of metabolites for methylation and acetylation.